Ch4 Interactions: Mastering Molecular Behavior
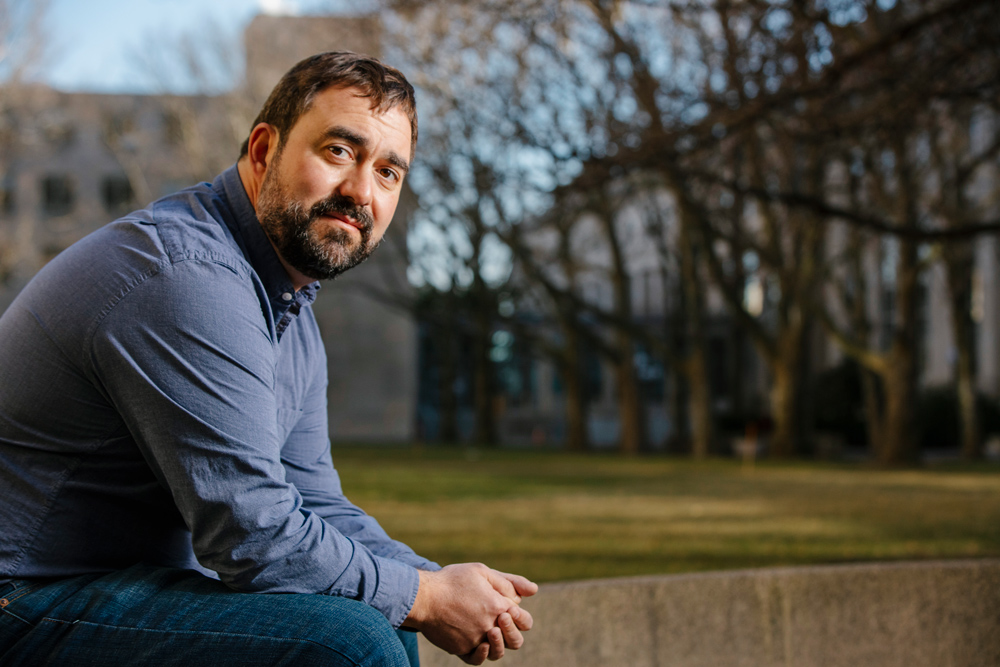
Understanding the intricacies of molecular interactions is crucial for grasping the behavior of matter at its most fundamental level. This realm, where physics, chemistry, and biology converge, holds the secrets to why substances exhibit specific properties and how they interact with each other. The study of molecular interactions delves into the heart of chemistry and materials science, providing insights into the formation of bonds, the solubility of substances, the viscosity of liquids, and the strength of materials.
At the core of molecular interactions are the forces that molecules exert on each other. These forces, which can be either attractive or repulsive, determine the physical and chemical properties of substances. The primary forces involved in molecular interactions include ionic bonds, covalent bonds, van der Waals forces (including dipole-dipole, dipole-induced dipole, and induced dipole-induced dipole interactions), and hydrogen bonds. Each type of force has a specific role, with some being stronger and more permanent, like covalent bonds, and others being weaker and more transient, such as van der Waals forces.
Ionic Bonds: The Electrostatic Attraction
Ionic bonds are formed between two atoms when one or more electrons are transferred from one atom to another, resulting in the formation of ions with opposite charges. The electrostatic attraction between these positively and negatively charged ions holds them together, creating a strong and rigid bond. This type of bonding is typical in salts and minerals, such as sodium chloride (NaCl), where sodium loses an electron to become a positively charged ion (Na+), and chlorine gains an electron to become a negatively charged ion (Cl-). The strength and directionality of ionic bonds contribute to the crystalline structure of ionic compounds and their high melting and boiling points.
Covalent Bonds: Sharing Electrons
Covalent bonds, on the other hand, are formed when atoms share one or more pairs of electrons to achieve a more stable electronic configuration. This sharing can occur between atoms of the same element, as in the case of oxygen (O2) or hydrogen (H2) molecules, or between different elements, as seen in water (H2O) or methane (CH4). Covalent bonds can be polar or non-polar, depending on the difference in electronegativity between the atoms involved. Polar covalent bonds, where the electrons are not shared equally, result in a molecule with a partial positive charge on one side and a partial negative charge on the other, leading to the molecule being polar.
Van der Waals Forces: The Weaker Attractive Forces
Van der Waals forces, named after Johannes Diderik van der Waals, are weaker than ionic and covalent bonds but play a crucial role in the physical properties of substances, such as melting and boiling points, and in the solubility of gases in liquids. These forces arise from the interactions between temporary dipoles (induced dipoles) in non-polar molecules or between permanent dipoles in polar molecules. They are responsible for the attraction between molecules of noble gases, which do not form ionic or covalent bonds, and contribute to the viscosity and surface tension of liquids.
Hydrogen Bonds: A Special Case of Dipole-Dipole Interaction
Hydrogen bonds are a special type of dipole-dipole interaction that occurs when a hydrogen atom bonded to a highly electronegative atom (such as oxygen, nitrogen, or fluorine) experiences attraction to another electronegative atom in a different molecule or within the same molecule if it is sufficiently distant. Hydrogen bonds are individually weak but collectively significant, playing a crucial role in the structure of water, proteins, and nucleic acids. They are responsible for many of the unique properties of water, such as its high boiling point and surface tension, and are essential for the folding and stability of biological macromolecules like DNA and proteins.
The balance between different types of molecular interactions is what gives each substance its unique set of physical and chemical properties. Understanding these interactions is key to predicting how substances will behave under various conditions and to designing new materials with specific properties.
Mastering Molecular Behavior: Applications and Implications
The ability to understand and predict molecular behavior based on the interactions between molecules has numerous practical applications. In materials science, it helps in the design of new materials with desired properties, such as strength, conductivity, or optical properties. In pharmaceuticals, understanding molecular interactions is crucial for drug design, where the goal is to create molecules that interact favorably with specific biological targets. In environmental science, knowledge of molecular interactions can help predict the fate and transport of pollutants in the environment and guide the development of strategies for remediation.
Furthermore, advancements in computational chemistry and molecular modeling have significantly enhanced our ability to study molecular interactions in detail. These tools allow for the simulation of molecular dynamics, providing insights into how molecules interact over time and under different conditions. This capability is invaluable for predicting the behavior of complex systems, designing new materials, and understanding biological processes at a molecular level.
Looking Ahead: The Future of Molecular Interactions Research
As research into molecular interactions continues to evolve, it is expected to unveil new insights into the behavior of matter at the molecular and atomic levels. The integration of machine learning and artificial intelligence with molecular modeling is poised to revolutionize the field, enabling faster and more accurate predictions of molecular properties and interactions. This could lead to breakthroughs in fields such as energy storage, where the design of more efficient batteries relies on understanding and optimizing the interactions between molecules.
Moreover, the study of molecular interactions is becoming increasingly interdisciplinary, drawing on concepts and techniques from physics, chemistry, biology, and materials science. This convergence of disciplines is expected to yield innovative solutions to some of the world’s most pressing challenges, including sustainable energy, environmental conservation, and human health.
Steps to Analyze Molecular Interactions
- Identify the Types of Bonds Involved: Determine if the interaction involves ionic, covalent, van der Waals, or hydrogen bonds.
- Consider the Electronegativity of Atoms: Assess how the difference in electronegativity affects the polarity of covalent bonds and the overall molecule.
- Evaluate the Molecular Structure: Use models or computational tools to visualize the molecule and predict how its shape influences its interactions.
- Assess the Role of Solvents: If applicable, consider how the solvent affects the interactions between molecules, including solvation effects and solvent polarity.
- Predict Physical and Chemical Properties: Based on the analysis of molecular interactions, predict properties such as boiling point, solubility, viscosity, and reactivity.
FAQ Section
What are the primary forces involved in molecular interactions?
+The primary forces involved in molecular interactions include ionic bonds, covalent bonds, van der Waals forces, and hydrogen bonds. Each type of force plays a distinct role in determining the physical and chemical properties of substances.
How do molecular interactions influence the properties of water?
+Molecular interactions, particularly hydrogen bonds, are responsible for many of water's unique properties, such as its high boiling point, surface tension, and ability to dissolve a wide variety of substances. These interactions also play a crucial role in the structure and function of biological molecules like proteins and DNA.
What role does understanding molecular interactions play in drug design?
+Understanding molecular interactions is crucial for drug design as it allows for the creation of molecules that can interact favorably with specific biological targets, such as proteins or nucleic acids. This interaction is key to the drug's efficacy and selectivity, minimizing side effects by ensuring the drug binds to the intended target.
In conclusion, molecular interactions are the foundation upon which the properties and behaviors of substances are built. By understanding the nature and strength of these interactions, scientists can predict and manipulate the characteristics of materials, design new drugs, and elucidate the intricacies of biological processes. As research continues to advance, the detailed study of molecular interactions will remain at the forefront of scientific inquiry, driving innovation and discovery across a wide range of disciplines.